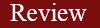
Ghrelin as a Promising Immunostimulant in Aquaculture: Mechanisms and Therapeutic Potential
Danielle Reyes *1, Mario P. Estrada 2, Rebeca Martínez *3
1 Metabolic Modifiers Project, Department of Animal Biotechnology,
Center for Genetic Engineering and Biotechnology, Havana, Cuba.
Center for Genetic Engineering and Biotechnology, Havana, Cuba.
2 Director of Agricultural Research, Center for Genetic Engineering and Biotechnology, Havana, Cuba; mario.pablo@cigb.edu.cu.
3 Metabolic Modifiers Project, Department of Animal Biotechnology,
Center for Genetic Engineering and Biotechnology, Havana, Cuba;
Center for Genetic Engineering and Biotechnology, Havana, Cuba;
* Correspondence: danielle.reyes@cigb.edu.cu
rebeca.martinez@cigb.edu.cu
ABSTRACT
Ghrelin, a peptide hormone originally known for its role in growth hormone secretion and appetite regulation, is a key immunomodulator in vertebrates, including fish. This hormone and its receptor (GHS-R) are widely expressed in immune cells—T cells, B cells, neutrophils, and macrophages—and tissues. In fish, Ghrelin modulates physiological processes (e.g., reproduction or energy balance) and improves immune defense mechanisms. Studies have demonstrated its ability to promote phagocytic activity, regulate cytokine production, and stimulate antimicrobial peptide production, enhancing resistance to pathogenic infections. Likewise, the development of ghrelin mimetics, like GHRP-6, with a wide range of functionalities, further increases the potential of their use in aquaculture, showing promising results in improving growth and immune responses in fish. Ghrelin and its analogs represent a promising alternative for boosting fish's immune response against pathogens. Thus, understanding Ghrelin's regulatory mechanisms in fish immunity may reveal practical applications for aquaculture practices, helping achieve fish welfare and sustainability.
Keywords: Ghrelin; immune system; fish; growth hormone secretagogue; cytokines; antimicrobial activity
INTRODUCTION
In 1996, a G-protein-coupled receptor was identified, GHS-R1a, back then considered an orphan receptor 1. The endogenous ligand of this receptor, Ghrelin (GHRL), was reported for the first time in 1999 in rats and human stomachs 2. This peptide hormone is mainly secreted from the stomach. Still, it's also expressed in other organs such as the brain, pituitary, placenta, ovaries, testes, kidneys, small intestine, pancreas, and lungs 3. Although its better-known functions are acting as a growth hormone (GH) secretagogue and regulating energy balance and food intake, it plays many roles in all body systems, such as improving cardiac performance, decreasing blood pressure, and inhibiting insulin release 4.
The immune and neuroendocrine systems are intrinsically linked in constant bidirectional communication 5, essential to maintaining homeostasis. The neuroendocrine system has multilevel modulatory properties that affect the functions of the immune system, contributing to both activation and suppression 6. The crosstalk between the immune system and the endocrine axis that controls appetite and metabolism has gained increasingly more interest. Recent studies have proved that Ghrelin can be crucial in this crosstalk 7, 8, 9, 10. Thus, this hormone is a central factor that regulates the immune response of various organisms.
This review focuses specifically on Ghrelin's role in fish immunology. Immune cells, like T cells, B cells, and neutrophils, express growth hormone (GH) and Ghrelin and have receptors for these hormones 11. Ghrelin, the expression of its receptor, and its mRNA are regulated during chronic inflammation, stress, and immune cells exposed to different pathogens 12. As a growth hormone secretagogue, in increasing GH secretion, Ghrelin modulates cytokine production, enhances B cell maturation and antibody production, and potentiates neutrophile adhesion and monocyte migration, besides having anti-apoptotic effects 13, 14, 15. As for its effects, Ghrelin attenuates septic shock, has anti-inflammatory effects, modulates phagocytosis, and promotes thymopoiesis 16, 17, 8. With more and more evidence gathering up on Ghrelin's influence on the immune response of non-mammalian vertebrates, including fish, the purpose of this article is to give an overview of the structure and expression of Ghrelin, its physiological functions, and the relationship between this orexigenic hormone and fish immune system.
Structure of Ghrelin and its receptor
Ghrelin is a 28-amino acid peptide (GSSFLSPEHQKAQQRKESKKPPAKLQPR), with slight variations in length and structure across species, mainly released in the stomach by oxyntic cells 18, 19. The genomic organization of the ghrelin gene (GHRL) was first described in humans 20. This gene comprises 3 introns and 4 exons and is located in chromosome 3p25-26 21. The goldfish ghrelin gene was the first non-mammalian vertebrate gene to be identified, which shows the same genomic organization described in humans 22. This peptide hormone is encoded by the ghrelin and obestatin prepropeptide (GHRL) gene and the ghrelin O-acyltransferase (GOAT) then acylates Ghrelin at Ser3 with octanoic acid 18,23. Once acylated, proghrelin is transferred into the Golgi apparatus and cut into mature ghrelin 24. There are two forms of Ghrelin in the body: acyl ghrelin and des-acyl ghrelin. The desacylated form is more abundant in blood (90%) than the acylated (10%) 25. However, des-acyl ghrelin can neither bind GHS-R nor exhibit GH-releasing activity, even though food intake is regulated by it to the same extent as ghrelin 26.
The growth hormone secretagogue receptor's gene is located on chromosome 3q26–q29 27. It is alternatively spliced and exists in two isoforms, namely GHS-R1a and GHS-R1b, in most vertebrates 28. The active ghrelin receptor, known as GHS-R1a, is a G-protein-coupled receptor with seven transmembrane domains; the GHS-R1b modulates the 1a receptor 17. This type 1b receptor is a COOH-terminal truncated form of the type 1a receptor and is pharmacologically inactive 29.
Expression of Ghrelin and GHS-R
Ghrelin expression is tissue-specific, with the highest levels in the stomach and brain 30. This hormone and its receptors are widely expressed in many brain regions, pituitary, intestine, kidney, thyroid gland, lung, heart, pancreatic islets, ovaries, testis, and sebaceous glands 4. Even though its better-known effects are being a GH secretagogue and playing an essential part in food intake regulation, it affects the central nervous, cardiovascular, reproductive, and immune systems 17.
Immune cells express both Ghrelin and its receptor. The study carried out by Hattori et al. shows that GHS-R and Ghrelin were expressed not only in B cells but also in T cells and neutrophils that did not express substantial GH transcripts, suggesting that ghrelin/GHS-R has unknown biological functions other than enhancing GH secretion in the immune system 11. Meanwhile, this hormone's expression was shown to be decreased in peritoneal macrophages during HFD intake, and this was associated with increased inflammatory cytokine production, although it exercised an increase in ghrelin expression in these macrophages and reduced inflammatory cytokine production 7. Ghrelin treatment modulates the secretion of polarizing cytokines of dendritic cells (DCs) during their maturation and thymic DCs 31, 32. The growth hormone secretagogue receptor's expression is more restricted than its ligand's. However, it is also present in immune cells. The first report of the presence of GHS-R in the immune system was in 2001, in human T cells, B cells, and neutrophils 11. Later on, macrophages and monocyte-derived dendritic cells have also been shown to express the growth hormone secretagogue receptor 33,34, 35,36.
Ghrelin across different species
Ghrelin is produced across the whole spectrum of vertebrates 37. This hormone has been identified in several species, including humans, pigs, rats, mice, eels, goldfish, and tilapia 38. Ghrelin remained a single-gene locus in all vertebrates 39. However, there are variations in length and structure between these groups.
In mammals, one form of ghrelin peptide and its receptor are present. This is not the case for non-mammalian vertebrates, where multiple forms of ghrelin and ghrelin receptors exist 37. For instance, Ghrelin's N-terminal 7 amino acids are conserved across teleosts, amphibians, reptiles, and mammals, but specific variations exist, such as the presence of Thr-3 in amphibians 40. In fish, Ghrelin's sequence seems to be conserved; however, slight differences have been identified. According to Zhong et al., the prepropeptide length ranges from 103 to 108 amino acids, and the similarities between the seven species in that study covered 35.00% to 89.32% 23. Accordingly, Wang et al. stated that, in bighead carp, the ghrelin gene encodes a protein of 106 amino acids 30. Cod ghrelin, however, has a longer precursor length (122 amino acids) compared to the 102-111 amino acids found in other species; it also contains specific amino acid variations, such as Thr-3, which is also seen in frog ghrelin, and valine as the second amino acid, similar to elasmobranchs 40.
The differences amongst vertebrate groups are also evident in Ghrelin's functions, although its effect as a growth hormone secretagogue seems to be conserved across vertebrates. In mammals like rodents and humans, Ghrelin is considered lypolitic, maintains body adiposity, and regulates glucose metabolism, and its effects in stimulating feeding are well known 41,42. In contrast, centrally injected Ghrelin inhibits food intake in neonatal chickens and may shift metabolic fuel preference from carbohydrates to lipids 43. On the other hand, evidence suggests the loss of the ghrelin gene entirely 44 in some avian species, like falcons. Ghrelin was also shown to decrease food intake in juvenile rainbow trout (Oncorhynchus mykiss) through the central anorexigenic corticotropin-releasing factor system, although in fish, it's known as a hormone that stimulates feeding 45,46. In non-mammalian vertebrates, Ghrelin stimulates reproductive functions 47. Fish ghrelin, specifically, stimulates the release of the luteinizing hormone 48. This is in contrast to its effect in mammalian species, where ghrelin treatment inhibits GnRH, LH, and FSH secretion at the hypothalamic and pituitary levels 49.
Physiological functions of Ghrelin in fish
Though initially identified for inducing an increased secretion of GH in the stomach, Ghrelin has a broad range of functions on all body systems. The administration of this hormone usually stimulates feeding and an increased body weight in mammals and non-mammals, except birds. Consequently, becoming the first peripheral hormone in being a strong appetite stimulant 46. It regulates sleep, gastric acid secretion and body temperature 29,50. It also exhibits hypothalamic activities that result in stimulation of prolactin (PRL) and ACTH secretion, negatively influences the pituitary-gonadal axis at both central and peripheral level, influences behavior, modulates pancreatic exocrine and endocrine function, and affects glucose levels 51. Likewise, evidence suggests Ghrelin's capacity to suppress inflammation 52. Amongst its various applications, it improves cardiovascular functions, higher brain functions and prevents neurodegenerative diseases 4.
In fish, Ghrelin regulates diverse physiological processes and influences locomotor activity. This hormone positively affects oocyte maturation, ovarian vitellogenesis, and the number of mature follicles of Barbus sharpeyi, while it suppresses reproduction when energy levels are low in Carassius auratus 53,54. Research also revealed that Ghrelin stimulates luteinizing hormone secretion from the pituitary cells of Cyprinus carpio and Carassius auratus 55,56. In 2019, a study proved for the first time the effects of an early stimulus of Ghrelin during the embryonic stage of zebrafish, where Ghrelin treated larvae showed higher swimming activity 57. More evidence of this hormone-modifying locomotor activity was gathered in goldfish (Carassius auratus) 58.
Similarly, Ghrelin modulates energy balance by regulating food intake, body weight, and glucose homeostasis 59. In juvenile Persian sturgeon (Acipenser persicus), ghrelin injection significantly increased GH production and high doses decreased cortisol levels, although there were not significant changes in prolactin levels 60. There's also evidence of its effect in the modulation of glucoregulatory machinery, glucose homeostasis in fish, and the regulation of insulin synthesis 61,62. Likewise, simultaneous ghrelin treatment counteracted the response to increased oleate levels, modulating hypothalamic fatty acid sensing 63. The study by Salmeron et al. confirms this role in regulating lipid metabolism, being an enhancer of lipid turnover in the adipose tissue of rainbow trout 64.
Ghrelin and fish immune system
Besides the previously mentioned effects of Ghrelin, it is a potent immunomodulator with many possible applications in the fish's immune system (Figure 1). This hormone is a natural antimicrobial and anti-inflammatory peptide, widely distributed in all body tissues and especially abundant in nonspecific immune organs 4. Immune system signaling may directly influence the metabolic axis and reciprocally via the expression of several common receptors and cytokine and hormonal ligands; changes in the energy balance and metabolism may exert potent effects on immune function and trafficking 28. Over the years, accumulating evidence highlights the importance of Ghrelin in regulating inflammation, apoptosis, cytokine production, and phagocytic activity, among other essential aspects of immune response 3,65–68. In mammalian models, for example, its anti-inflammatory effects have been described 69.

Figure 1. Multifaceted immunomodulatory roles of Ghrelin in teleost fish: Mechanisms and functional outcomes. Ghrelin, acting primarily through its receptor GHS-R, plays diverse roles in fish immunity. It enhances phagocytic activity via the JAK2/STAT5 signaling pathway and promotes reactive oxygen species (ROS) production, aiding pathogen elimination. It also regulates cytokine production, including TNF-α, IFN-γ, and TGF-β, contributing to immune modulation. Additionally, Ghrelin exhibits direct antimicrobial activity through charge-dependent microbial binding and inducing antimicrobial peptide (AMP) production via the NF-κB pathway. Finally, Ghrelin supports lymphocyte development, especially T cell proliferation, through the GH/GHR axis, emphasizing its potential as an immunostimulant in aquaculture.
Ghrelin and phagocytosis
Phagocytosis is a defensive reaction against infection and invasion of the body by foreign substances. In the immune system, phagocytosis is a major mechanism to remove pathogens and/or cell debris 70. It can interconnect the innate and adaptative immune responses since the pathogen's processing by professional phagocytes is a fundamental stage for antibody production. Evidence shows that Ghrelin is capable of promoting phagocytic activity 71. However, administering 20 μg/kg of Ghrelin via intraperitoneal injection reduced the increased phagocytic activity induced by acute cold restraint stress (ACRS) in rodents 72. Additionally, mouse mitochondrial macrophages treated with a 100 ng/mL dose showed a metabolic shift towards oxidative metabolism, which involves ultrastructural and functional protection from inflammatory signals that induce mitochondrial damage 73. This contrast could be due to dose differences and/or stress conditions, modulating overactivation to prevent immunopathology.
In fish, phagocytic activity is essential as a defense mechanism. As a growth hormone secretagogue, Ghrelin might increase phagocytic activity by increasing GH secretion. Evidence suggests GH's capacity to enhance phagocytosis 74,75. In a study published in 2020, evaluating Ghrelin's modulation of the immune response against Aeromonas hydrophila in juvenile hybrid tilapia, this treatment, via intraperitoneal injection, showed increased phagocytic activity by increasing ROS activity at 5h with doses of 20 ng and 200 ng diluted in 0.1 mL of PBS, being ROS the main mediator of phagocytosis 16. In an in vitro study in Oncorhynchus mykiss, using Ghrelin at 10 nM, this orexigenic hormone enhances superoxide production in leukocytes, promoting phagocytosis by binding to GHS-R, activating G-protein-coupled pathways to enhance oxidase-dependent superoxide production while also up-regulating autocrine GH secretion 76, which could amplify immune responses via JAK2/STAT5 signaling.
These studies show that Ghrelin can directly or indirectly regulate phagocytosis, promoting or decreasing it according to the organism's current needs. Interestingly, Ghrelin enhances phagocytic activity in fish, while the results vary in mammals.
Ghrelin and cytokine production
Cytokines are vital mediators that oversee and regulate immune and inflammatory responses via complex networks 77. Regulating cytokine production is essential for organisms to ensure a balanced immune response, preventing underreaction to pathogens and overreaction. Previous studies have stated that acyl-ghrelin suppresses proinflammatory cytokine expression and/or production 12,78. Ghrelin reduces the secretion of proinflammatory cytokines such as IL-6 and TNF-α, which could be overexpressed in inflammatory processes 65. In a study by Beynon et al. ghrelin (1, 10, 100 nM) attenuated IL-6 secretion in an LPS challenge in mid-brain dopaminergic neurons, suggesting possible applications in protecting against dopaminergic SN nerve cell damage 79. In 2021, this hormone appeared as an alternative against the cytokine storm produced by SARS‑CoV2 infection, mitigating the uncontrolled cytokine production by up-regulating PPARγ and down-regulating NF-κB expression 80. Ghrelin treatment also suppressed Th1 (IL-2 and IFN-g) and Th2 (IL-4 and IL-10) cytokines mRNA expression in mice 81.
Fish cytokines are similar to those found in higher vertebrate groups, such as interleukin-1β 82. Against an Aeromonas hydrophila infection in hybrid tilapia, Ghrelin (2.0 ng, 20 ng, and 200 ng diluted in 0.1 mL of PBS) showed regulatory effects on the expression of IL-1β, decreased up-regulated TGF-β expression to normal levels and increased TNF-α expression by up-regulating GH levels 16. Similar results were obtained in 2011 evaluating GH treatment's effect on TNF-α expression in Oreochromis niloticus, showing an increased expression in immune organs with an intraperitoneal injection of 2 mg/g of body weight 83. In a study with different stimuli (lipopolysaccharide, phytohemagglutinin, or imiquimod), an increased ghrelin expression downregulated the production of cytokines, such as interleukin (IL)-1β, IL-6, and tumor necrosis factor (TNF)- α 84. A study by Han et al. showed Ghrelin, using a dose of 1.25 ng/ µL, regulates cytokine production in fish by a combination of inhibiting certain proinflammatory cytokines (IL-6, TNF-α), inducing others and modulating immune-related gene expressions via two routes: directly mediating cytokine expression and indirectly altering downstream signaling of multifunctional bioregulators (TNF-α, IFN-γ, and TGF-β), simultaneously 8. These mechanisms collectively contribute to controlling inflammation and maintaining immune system balance in fish. This shows Ghrelin's prospective uses as a treatment to regulate inflammation via downregulating proinflammatory cytokines and up-regulating regulatory cytokines, acting similarly in mammals and fish.
Ghrelin regulation of lymphocyte development
The thymus is essential for developing, selecting, and maintaining the peripheral T cell pool possessing a broad spectrum of TCR specificities 85. In a study of old mice injected intraperitoneally with 2 nM of Ghrelin, the treatment did not increase peripheral lymphocytes, but they did exhibit a statistically significant increase in thymic cellularity and differentiation 86. Dixit et al. showed induced thymopoiesis during aging in mice with ghrelin treatment (1.25 μg/h for 2 weeks); the interplay between ghrelin and ghrelin receptor interactions in the thymic compartment and bone marrow stem cell niches leads to rejuvenation of thymic output and improved T cell repertoire in old animals 35. Ghrelin also plays an important role in reestablishing the proliferation of CD4+ T cells and serves as a promising therapeutic agent in sepsis in humans 87. Likewise, this hormone modulates Th effector cells in the gut, controlling proliferation and inducing apoptosis 88. By increasing GH secretion, Ghrelin might indirectly induce this hormone's effects in immune cells, like enhancing B cell development and antibody production 3. This shows Ghrelin's capacity to regulate immune cell development and proliferation, whether its effect is direct or indirect. When evaluating the impact of somatotrophic axis (GH/GHR) double transgenesis on structural and molecular aspects of the zebrafish immune system, there was a decrease in CD3+ and CD4+ T cells, showing the relevance of this axis for fish immune response 89 and, therefore, the importance of GH on fish lymphocyte development. Further research is needed to understand better how Ghrelin can regulate these aspects of the immune system in fish.
Ghrelin's antimicrobial activity
Ghrelin can inhibit the growth of microorganisms that could harm the organism, also known as antimicrobial activity. Ghrelin is a natural antimicrobial and anti-inflammatory peptide, widely distributed in all body tissues and especially abundant in the proximity to physical barriers such as the stomach, gut, and skin 90. This hormone shows antimicrobial properties by inhibiting the growth of Helicobacter pylori 91. Both acyl ghrelin and des- acyl ghrelin possess similar antimicrobial activity against Escherichia coli and Pseudomonas aeruginosa. Ghrelin concentrations equal to, or greater than, 12.5 μg/ml showed significant bacterial killing effects toward these two Gram-negative bacteria, and antimicrobial activity could be derived from AMPs that facilitate their charge-dependent binding to bacteria 92. In rainbow trout, Ghrelin modulates the expression of antimicrobial peptides' genes; this may result from a combination of ghrelin-mediated functional perturbations of GHS and NF-κB signaling pathways in RT-HKD cells 8, which could lead to increased antimicrobial activity. Ghrelin is also a prospective antimicrobial peptide that can decrease the activity of Aeromonas hydrophila 16. This proves Ghrelin's capacity to modulate antimicrobial activity, whether by acting as an antimicrobial peptide or by regulating the expression of genes related to antimicrobial peptides. These results, gathered with those of previous sections, show that Ghrelin can regulate fish's immune system. This hormone exhibits species-specific immunomodulatory effects, enhancing phagocytic activity, regulating cytokine production, and boosting antimicrobial defenses in teleosts (Table 1).
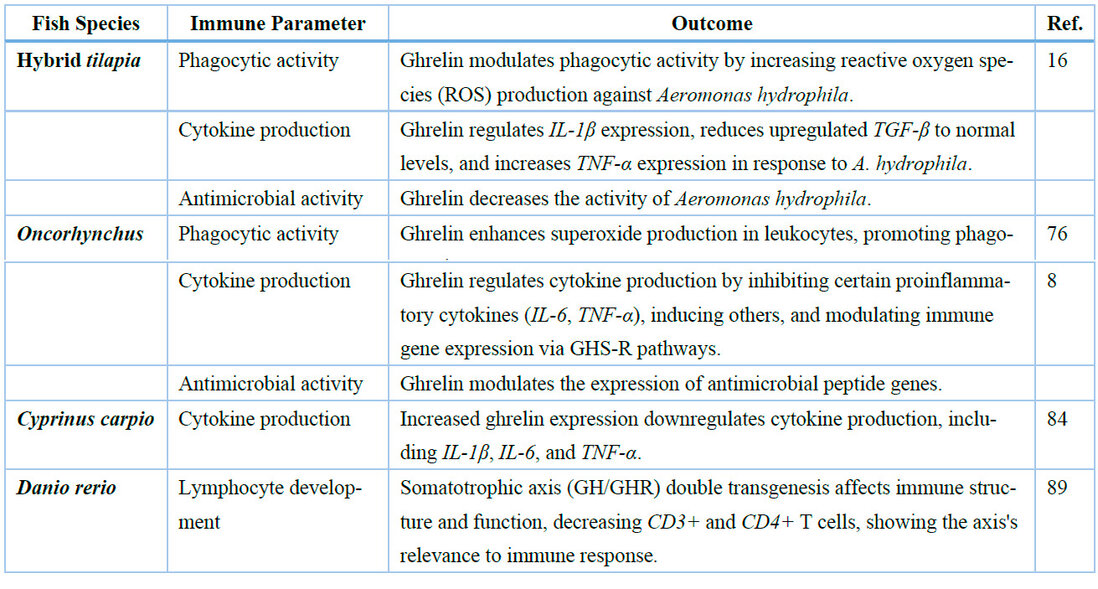
Table 1. Comparative analysis of ghrelin-mediated immune responses across teleost species: Phagocytic activity, cytokine production, and antimicrobial defense.
Molecular mechanisms of ghrelin’s immunomodulatory effects
As previously stated here, the immunomodulatory effects of Ghrelin have been extensively documented in fish and other vertebrates, but there are still gaps in knowledge regarding the molecular pathways underlying such effects. The body of evidence is growing, but there is still a lack of complete understanding of these mechanisms, particularly in aquatic species. However, some of these signaling cascades have been identified to some extent (Figure 2).
NF-κB Pathways
The NF-κB signaling pathway plays a crucial role in regulating the transcription of cytokine-encoding genes 80. Likewise, the production of AMPs is often regulated by Toll-like receptors and NOD-like receptors mediated by NF-κB signaling pathways. Notably, Ghrelin has been demonstrated to modulate NF-κB signaling across species, suggesting a conserved immunoregulatory mechanism 8.
NF-κB activity is primarily controlled by a dynamic interplay between its inhibitors (IκB) and IκB kinases (IKK), enabling adaptation to the prevailing circumstances and preventing excessive immune responses 93. Once activated, the IκB kinase complex phosphorylates IκB proteins, leading to their degradation and NF-κB nuclear translocation for inducing gene expression; Ghrelin suppresses NF-κB by inhibiting NOD2-Rip2 signaling, an upstream activator of this pathway 80. Meanwhile, the Ghrelin-GH-IGF-I growth axis up-regulates the expression of NF-kB 94. This shows Ghrelin's dual role in modulating immune responses according to the organism's needs.
Nrf2-Keap1/ARE pathway
ROS is important in stress perception, integration of diverse stress-responsive signaling networks, and activation of animal defense mechanisms, frequently occurring during inflammation in fish 95. Nrf2, regulated by Keap1, is an important endogenous modulator of ROS overproduction, and Ghrelin has been shown to enhance Nrf2 expression and reduce oxidative damage 80. In the Nrf2-Keap1/ARE pathway, the nuclear transcription factor Nrf2 translocates into the nucleus to initiate the transcription of antioxidant genes, thereby reducing reactive oxygen species (ROS)-induced cellular damage and maintaining the organism's oxidative-antioxidative equilibrium 96.
JAK2/STAT5 pathway
JAK2/STAT5 signaling intervenes in several immune processes in vertebrates, including respiratory bursts and the expression of proinflammatory cytokines 97. Ghrelin can act indirectly through the JAK2/STAT5 pathway by inducing an enhanced GH secretion. GH can activate Janus Kinase 2 (JAK2)/transcription factors STAT5 94. Ghrelin binds to its receptor, growth hormone secretagogue receptor 1a (GHSR1a), which is widely expressed in central systems and peripheral organs and stimulates the production of the growth hormone 65. The JAK2/STAT5 pathway is the principal GH signaling mechanism, leading to transactivation and/or repression of target genes, including IGF-1 98. JAK2 initiates signaling upon cytokine receptor activation through auto/transphosphorylation, creating phosphotyrosine docking sites for STAT transcription factors, which SOCS proteins can inhibit. If there's no suppression of JAKS2, STATs are then phosphorylated by JAKs, forming dimers that translocate to the nucleus to regulate target gene expression 99. Therefore, Ghrelin's ability to modulate immune response is shown by increasing GH secretion.
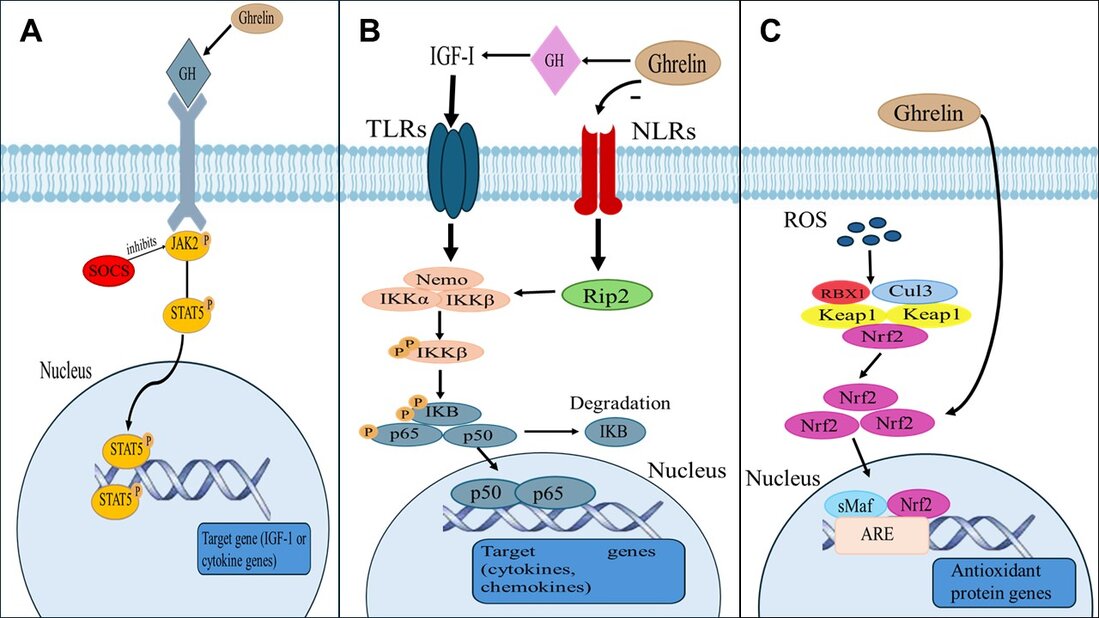
Figure 2. Ghrelin modulates key immune signaling pathways in fish. (A) JAK2/STAT5 pathway: Ghrelin, via growth hormone (GH) stimulation, activates the JAK2/STAT5 axis, leading to phosphorylation of STAT5 and transcription of immune-regulatory genes such as IGF-1 and cytokines. SOCS proteins negatively regulate this pathway, which inhibits JAK2 activity to maintain homeostasis. (B) NF-κB pathway: Ghrelin influences the balance between Toll-like receptors (TLRs) and NOD-like receptors (NLRs), modulating downstream signaling cascades. TLR activation leads to phosphorylation of IKKβ and degradation of IκB, allowing NF-κB (p65/p50) to translocate to the nucleus and induce expression of cytokines and chemokines. Ghrelin also impacts IGF-I levels, linking growth and immune signaling. (C) Nrf2-Keap1/ARE pathway: In response to oxidative stress and reactive oxygen species (ROS), Ghrelin promotes the dissociation of Nrf2 from the Keap1-Cul3 complex. Free Nrf2 translocates into the nucleus where, together with small Maf proteins, it binds to the antioxidant response element (ARE) and drives the expression of antioxidant and cytoprotective genes, reinforcing cellular defense mechanisms.
GHRP-6 and its effects in fish immunity as a mimetic of ghrelin
In 1976, Bowers et al. showed the functionality of met-encephalin-derived forms as promoters of GH secretion in rodent's pituitary cells 100. Even though their signaling pathways were unknown then, this research paved the way for the future development of peptidic growth hormone secretagogues. GHRP-6 (His-(D-Trp)-Ala-Trp-(D-Phe)-Lys-NH2) is a mimetic of Ghrelin. The peptide was developed in 1984 and proved its possible applications by increasing GH levels with relatively low doses 101.
GHRP-6 reduces the rate of apoptosis in cerebellar cells of aged rats 102. It can also attenuate doxorubicin (Dox) cytotoxicity and maintain antioxidant cellular defense 103. On shrimps Litopenaeus vannamei, GHRP-6 treatment increased total hemocyte counts, a potential indicator of crustacean immune response 104. This peptide also possesses anti-inflammatory, antioxidant, and cytoprotective properties 105.
Studies carried out by Martinez et al. (2016) demonstrated that GHRP-6 (0.1 μg/gram of body weight) treatment can increase lectin titters and the number of intestinal intraepithelial lymphocytes in Oreochromis sp. larvae via oral administration 106. Likewise, GHRP-6 (0.2 μg/gram of body weight) affects the positive regulation of the transcription levels of three piscidin-like antimicrobial peptides (Oreochromicin I, II and III) and granzyme in a tissue-dependent manner, as well as an improvement in the antimicrobial activity of the serum and the decrease in the bacterial load of Pseudomonas aeruginosa, when injected intraperitoneally 107. Given that dietary supplementation with GHRP-6 (500 μg GHRP-6/kg of feed) improves growth performance in juvenile gilthead sea bream 108, exploring its immunostimulatory potential via oral administration could be of significant interest.
Other analogs of Ghrelin may also serve as treatments to enhance the immune system, growth, and other physiological functions. According to a previous study by Martinez et al. (2016), therapy with A228 significantly increased the body weight of tilapia larvae and enhanced superoxide production in tilapia peripheral blood leukocyte cultures 109. Moreover, the peptide A233 also induces GH secretion, induces superoxide production in tilapia head–kidney leukocyte culture and has antiviral activity 110,111.
Future perspectives
Although significant progress has been made in understanding Ghrelin's immunomodulatory roles, key gaps remain in its mechanistic and comparative biology. The influence of Ghrelin in fish lymphocyte development needs further research, as do the molecular mechanisms behind Ghrelin's effects in teleost immunity. Most studies are limited to a single species. A broader focus is needed, especially considering species-specific effects due to different environments and, therefore, possibly, different pathogenic threats. Furthermore, extrapolations from the predominant research in mammals to similar research in fish could be complicated due to evolutionary and physiological divergence. Rather than direct cross-species comparisons, a robust evolutionary framework could better contextualize these differences.
Future studies regarding fish must further clarify Ghrelin's molecular mechanisms regulating immune responses in diverse species. Comparative analyses across taxa might uncover species-specific adaptations, therefore, making tailored ghrelin-based treatments that could be more promising for aquaculture. Direct research into Ghrelin's effects on leukocyte function (e.g., antibody production) and GHS-R1a knockout models (e.g., CRISPR-Cas9 in zebrafish) could clarify receptor-specific versus GH-independent immune modulation, complementing current findings on phagocytosis and cytokine regulation. Transcriptomic profiling of immune cells exposed to acyl/des-acyl ghrelin may reveal novel pathways (e.g., NF-κB, JAK-STAT), while cross-species comparisons (e.g., tilapia vs. gar or tilapia vs. mice) could assess evolutionary conservation of these mechanisms. This could prove conserved immunomodulatory functions of Ghrelin across vertebrates, particularly its anti-inflammatory and antimicrobial properties in fish, and offer translational insights for human medicine—potentially informing therapies for inflammatory diseases, sepsis, or immune aging. Furthermore, its role in lymphocyte development and stress adaptation could bridge comparative immunology and regenerative medicine, where Ghrelin's tissue-protective effects are increasingly explored.
Given aquaculture's challenges, larval immune studies and stress experiments (e.g., Ghrelin's role in crowding-induced immunosuppression) would clarify its potential to enhance resilience, particularly under climate change pressures. Finally, quantifying ghrl expression in the wild versus farmed populations and its possible relation to immune parameters could uncover domestication impacts on immune function. Additionally, research should explore more the applications of novel ghrelin mimetics with improved stability and targeted effects, like GHRP-6. Such multidisciplinary approaches, spanning molecular, ecological, and industry-relevant frameworks, would advance Ghrelin's application as a sustainable alternative to antibiotics.
Future applications in aquaculture should prioritize standardized protocols for ghrelin administration, including intraperitoneal injections (20–200 ng/g body weight, as effective in tilapia), oral delivery similar to that of GHRP-6-supplemented feed (500 μg GHRP-6/kg of feed), or immersion treatments for larvae. Combining ghrelin analogs with probiotics or vaccines could also result in even better results by enhancing fish's immune system. Industry-scale adoption will require cost-effective production of stable mimetics (e.g., GHRP-6), slow-release formulations, and regulatory approval. Pilot trials in commercial species (e.g., salmon, shrimp) must validate these approaches under farm conditions to bridge lab findings to aquaculture in fish farms.
CONCLUSIONS
The production of healthy fish is essential for aquaculture profitability. As the aquaculture industry grows, so do the possibilities for the emergence and spread of infectious diseases. Pathogens cause an estimated annual loss of 6 trillion USD to the aquaculture sector worldwide 112. The most common treatment for these pathogens is antibiotics. However, antibiotic resistance has become an increasingly pressing matter 113. This searches for alternative therapies to enhance fish immune response, such as Ghrelin, which is a crucial need.
Ghrelin is a multifunctional peptide hormone, first identified for regulating growth hormone release and appetite. Recent research has revealed its critical part in vertebrate immune system modulation. The wide expression across tissues and receptor presence in immune cells underscore its immunomodulatory capacity. This hormone orchestrates diverse immune functions—from phagocytosis and cytokine balance to thymic development and antimicrobial defense—positioning it as a promising immunotherapeutic agent 3. This hormone regulates physiological processes in fish while dynamically shaping immune responses 8,16,77,85. Its effects include augmented phagocytosis, precise cytokine regulation, and antimicrobial peptide synthesis, stimulating host defenses. Its anti-inflammatory actions further highlight a therapeutic potential for immune enhancement. Additionally, ghrelin mimetics (e.g., GHRP-6, A233, A228) could offer an alternative to antibiotics used for aquaculture. For instance, GHRP-6 improves growth metrics, immune performance, and pathogen resistance, acting as both a growth promoter and immunostimulant 106-108.
The ghrelin-immune interplay in fish demands deeper research to unlock its full therapeutic value. Harnessing Ghrelin and its analogs could revolutionize sustainable aquaculture by optimizing fish health and productivity, presenting itself as a sustainable alternative to antibiotics against pathogenic infections. Therefore, considering the current worldwide antibiotic resistance crisis, reducing significant economic losses in the industry and becoming a possible treatment. As evidence accumulates, ghrelin immunology will probably remain an ever-growing area of research, yielding innovations for aquaculture efficiency and animal welfare.
Author Contributions: Methodology: Danielle Reyes and Rebeca Martinez. Investigation: Danielle Reyes and Rebeca Martinez. Data curation: Danielle Reyes and Rebeca Martinez. Writing: Danielle Reyes and Rebeca Martinez. Visualization: Danielle Reyes, Rebeca Martinez and Mario P. Estrada . Supervision: Rebeca Martinez. Project administration: Rebeca Martinez. Resources: Danielle Reyes, Rebeca Martinez y Mario P. Estrada
Funding: This research received no external funding.
Institutional Review Board Statement: Not applicable.
Informed Consent Statement: Not applicable.
Conflicts of Interest: The authors declare no conflict of interest.
Institutional Review Board Statement: Not applicable.
Informed Consent Statement: Not applicable.
Conflicts of Interest: The authors declare no conflict of interest.
REFERENCES
1. Howard AD, Feighner SD, Cully DF, Arena JP, Liberator PA, Rosenblum CI, et al. A receptor in pituitary and hypothalamus that functions in growth hormone release. Science. 1996;273(5277):974-7. doi:10.1126/science.273.5277.974
2. Kojima M, Hosoda H, Date Y, Nakazato M, Matsuo H, Kangawa K. Ghrelin is a growth-hormone-releasing acylated peptide from stomach. Nature. 1999;402(6762):656-60. doi:10.1038/45230
3. Hattori N. Expression, regulation and biological actions of growth hormone (GH) and Ghrelin in the immune system. Growth Horm IGF Res. 2009;19(3):187-97. doi:10.1016/j.ghir.2008.12.001
4. Akalu Y, Molla MD, Dessie G, Ayelign B. Physiological effect of Ghrelin on body systems. Int J Endocrinol. 2020;2020:1385138. doi:10.1155/2020/1385138
5. Taub DD. Novel connections between the neuroendocrine and immune systems: the ghrelin immunoregulatory network. Vitam Horm. 2007;77:325-46. doi:10.1016/S0083-6729(06)77014-5
6. Miyake S. Mind over cytokines: crosstalk and regulation between the neuroendocrine and immune systems. Clin Exp Neuroimmunol. 2012;3(1):1-15. doi:10.1111/j.1759-1961.2011.00023.x
7. Chowen JA, Argente J. Ghrelin: a link between energy homeostasis and the immune system. Endocrinology. 2017;158(7):2077-81. doi:10.1210/en.2017-00350
8. Han YC, Leaman DW, Shepherd BS. Ghrelin modulates differential expression of genes relevant to immune activities and antimicrobial peptides in primary head kidney cells of rainbow trout (Oncorhynchus mykiss). Animals. 2023;13(10):1683. doi:10.3390/ani13101683
9. Muñoz-Flores C, Roa FJ, Saavedra P, Fuentealba P, Starck MF, Ortega L, et al. Immunomodulatory role of vasoactive intestinal peptide and Ghrelin in Oncorhynchus mykiss. Heliyon. 2023;9(12):e23098. doi:10.1016/j.heliyon.2023.e23098
10. Zhu J, Zhao H, Aierken A, Zhou T, Menggen M, Gao H, et al. Ghrelin is involved in regulating the progression of Echinococcus granulosus-infected liver lesions through suppression of immunoinflammation and fibrosis. PLoS Negl Trop Dis. 2024;18(10):e0012587. doi:10.1371/journal.pntd.0012587
11. Hattori N, Saito T, Yagyu T, Jiang BH, Kitagawa K, Inagaki C. GH, GH receptor, GH secretagogue receptor, and ghrelin expression in human T cells, B cells, and neutrophils. J Clin Endocrinol Metab. 2001;86(9):4284-91. doi:10.1210/jcem.86.9.7866
12. Baatar D, Patel K, Taub DD. The effects of Ghrelin on inflammation and the immune system. Mol Cell Endocrinol. 2011;340(1):44-58. doi:10.1016/j.mce.2011.04.019
13. Poudel SB, Dixit M, Neginskaya M, Nagaraj K, Pavlov E, Werner H, et al. Effects of GH/IGF on the aging mitochondria. Cells. 2020;9(6):1384. doi:10.3390/cells9061384
14. Noh JY, Herrera M, Patil BS, Tan XD, Wright GA, Sun Y. The expression and function of growth hormone secretagogue receptor in immune cells: a current perspective. Exp Biol Med. 2022;247(24):2184-91. doi:10.1177/15353702221121635
15. Reis MD, Veneziani LP, Porto FL, Lins MP, Mendes-da-Cruz DA, Savino W. Intrathymic somatotropic circuitry: consequences upon thymus involution. Front Immunol. 2023;14:1108630. doi:10.3389/fimmu.2023.1108630
16. Han Z, Zhou Y, Zhang X, Yan J, Xiao J, Luo Y, et al. Ghrelin modulates the immune response and increases resistance to Aeromonas hydrophila infection in hybrid tilapia. Fish Shellfish Immunol. 2020;98:100-8. doi:10.1016/j.fsi.2020.01.006
17. Mathur N, Mehdi SF, Anipindi M, Aziz M, Khan SA, Kondakindi H, et al. Ghrelin as an anti-sepsis peptide: review. Front Immunol. 2021;11:610363. doi:10.3389/fimmu.2020.610363
18. Yanagi S, Sato T, Kangawa K, Nakazato M. The homeostatic force of Ghrelin. Cell Metab. 2018;27(4):786-804. doi:10.1016/j.cmet.2018.02.008
19. De Vriese C, Delporte C. Ghrelin: a new peptide regulating growth hormone release and food intake. Int J Biochem Cell Biol. 2008;40(8):1420-4. doi:10.1016/j.biocel.2007.04.020
20. Wajnrajch MP, Ten IS, Gertner JM, Leibel RL. Genomic organization of the human ghrelin gene. Int J Disabil Hum Dev. 2000;1(4):231-4. doi:10.1515/IJDHD.2000.1.4.231
21. Unniappan S, Peter RE. Structure, distribution and physiological functions of Ghrelin in fish. Comp Biochem Physiol A Mol Integr Physiol. 2005;140(4):396-408. doi:10.1016/j.cbpb.2005.02.011
22. Unniappan S, Lin X, Cervini L, Rivier J, Kaiya H, Kangawa K, et al. Goldfish ghrelin: molecular characterization of the complementary deoxyribonucleic acid, partial gene structure and evidence for its stimulatory role in food intake. Endocrinology. 2002;143(10):4143-6. doi:10.1210/en.2002-220644
23. Zhong H, Hu Y, Yu F. A review on Ghrelin and fish reproduction. Reprod Breed. 2021;1(2):128-35. doi:10.1016/j.repbre.2021.07.004
24. Cornejo MP, Mustafá ER, Barrile F, Cassano D, De Francesco PN, Raingo J, et al. The intriguing ligand-dependent and ligand-independent actions of the growth hormone secretagogue receptor on reward-related behaviors. Neurosci Biobehav Rev. 2021;120:401-16. doi:10.1016/j.neubiorev.2020.10.017
25. Kurowska P, Mlyczynska E, Rak A. Effect of Ghrelin on the apoptosis of various cells. A critical review. J Physiol Pharmacol. 2019;70(1):1-12. doi:10.26402/jpp.2019.1.02
26. Sato T, Nakamura Y, Shiimura Y, Ohgusu H, Kangawa K, Kojima M. Structure, regulation and function of Ghrelin. J Biochem. 2012;151(2):119-28. doi:10.1093/jb/mvr134
27. Kissebah AH, Sonnenberg GE, Myklebust J, Goldstein M, Broman K, James RG, et al. Quantitative trait loci on chromosomes 3 and 17 influence phenotypes of the metabolic syndrome. Proc Natl Acad Sci USA. 2000;97(26):14478-83. doi:10.1073/pnas.97.26.14478
28. Dixit VD, Taub DD. Ghrelin and immunity: a young player in an old field. Exp Gerontol. 2005;40(11):900-10. doi:10.1016/j.exger.2005.09.003
29. Kojima M, Kangawa K. Ghrelin: structure and function. Physiol Rev. 2005;85(2):495-522. doi:10.1152/physrev.00012.2004
30. Wang P, Fu J, Luo M, Shi X, Zhu W, Wang L, et al. Molecular cloning and expression characteristics of ghrl and ghsr genes in bighead carp (Hypophthalmichthys nobilis). Aquac Fish. 2024;9:100-8. doi:10.1016/j.aaf.2023.12.001
31. Orlova EG, Shirshev SV, Loginova OA. Leptin and Ghrelin regulate dendritic cell maturation and dendritic cell induction of regulatory T-cells. Dokl Biol Sci. 2015;462:171-4. doi:10.1134/S001249661503014X
32. Pereira JA, Silva FC, de Moraes-Vieira PM. The impact of Ghrelin in metabolic diseases: an immune perspective. J Diabetes Res. 2017;2017:4527980. doi:10.1155/2017/4527980
33. Lin L, Lee JH, Buras ED, Yu K, Wang R, Smith CW, et al. Ghrelin receptor regulates adipose tissue inflammation in aging. Aging. 2016;8(1):178-91. doi:10.18632/aging.100888
34. Lee JH, Fang C, Li X, Wu CS, Noh JY, Ye X, et al. GHS-R suppression in adipose tissues protects against obesity and insulin resistance by regulating adipose angiogenesis and fibrosis. Int J Obes. 2021;45(7):1565-75. doi:10.1038/s41366-021-00820-7
35. Dixit VD, Yang H, Sun Y, Weeraratna AT, Youm YH, Smith RG, et al. Ghrelin promotes thymopoiesis during aging. J Clin Invest. 2007;117(10):2778-90. doi:10.1172/JCI30248
36. Orlova EG, Shirshev SV. Ghrelin regulation of in vitro human monocyte functional activity. Dokl Biol Sci. 2010;434(1):368-70. doi:10.1134/S0012496610050200
37. Kaiya H, Kangawa K, Miyazato M. Molecular evolution of GPCRs: ghrelin/ghrelin receptors. J Mol Endocrinol. 2014;52(3):T87-100. doi:10.1530/JME-13-0175
38. Korbonits M, Goldstone AP, Gueorguiev M, Grossman AB. Ghrelin—a hormone with multiple functions. Front Neuroendocrinol. 2004;25(1):27-68. doi:10.1016/j.yfrne.2004.03.002
39. Tine M, Kuhl H, Teske PR, Tschöp MH, Jastroch M. Diversification and coevolution of the ghrelin/growth hormone secretagogue receptor system in vertebrates. Ecol Evol. 2016;6(8):2516-35. doi:10.1002/ece3.2057
40. Kaiya H, Miyazato M, Kangawa K. Recent advances in the phylogenetic study of Ghrelin. Peptides. 2011;32(11):2155-74. doi:10.1016/j.peptides.2011.04.027
41. Heppner KM, Piechowski CL, Müller A, Ottaway N, Sisley S, Smiley DL, et al. Both acyl and des-acyl ghrelin regulate adiposity and glucose metabolism via central nervous system ghrelin receptors. Diabetes. 2013;63(1):122-31. doi:10.2337/db13-0414
42. Li Z, Xu G, Qin Y, Zhang C, Tang H, Yin Y, et al. Ghrelin promotes hepatic lipogenesis by activation of mTOR-PPARγ signaling pathway. Proc Natl Acad Sci USA. 2014;111(36):13163-8. doi:10.1073/pnas.1411571111
43. Geelissen SME, Swennen Q, Geyten SV, Kühn ER, Kaiya H, Kangawa K, et al. Peripheral Ghrelin reduces food intake and respiratory quotient in chicken. Domest Anim Endocrinol. 2006;30(2):108-16. doi:10.1016/j.domaniend.2005.06.005
44. Seim I, Jeffery PL, Herington AC, Chopin LK. Comparative analysis reveals loss of the appetite-regulating peptide hormone ghrelin in falcons. Gen Comp Endocrinol. 2015;216:98-102. doi:10.1016/j.ygcen.2014.11.016
45. Jönsson E, Kaiya H, Björnsson BT. Ghrelin decreases food intake in juvenile rainbow trout (Oncorhynchus mykiss) through the central anorexigenic corticotropin-releasing factor system. Gen Comp Endocrinol. 2010;166(1):39-46. doi:10.1016/j.ygcen.2009.11.001
46. Jönsson E. The role of Ghrelin in energy balance regulation in fish. Gen Comp Endocrinol. 2013;187:79-85. doi:10.1016/j.ygcen.2013.03.013
47. Izzo G, Ferrara D, Napolitano F, Crispo AA, d’Istria M, Aniello F, et al. Identification of a cDNA encoding for Ghrelin in the testis of the frog Pelophylax esculentus and its involvement in spermatogenesis. Comp Biochem Physiol A Mol Integr Physiol. 2011;158(4):367-73. doi:10.1016/j.cbpa.2010.09.005
48. Dar SA, Mattoo S, Srivastava PP. Ghrelin and its role in reproductive physiology of fish. In: Recent updates in molecular endocrinology and reproductive physiology of fish. Springer; 2021. p. 171-9. doi:10.1007/978-981-15-8369-8_12
49. Dupont J, Maillard V, Coyral-Castel S, Ramé C, Froment P. Ghrelin in female and male reproduction. Int J Pept. 2010;2010:158102. doi:10.1155/2010/158102
50. Hashimoto H, Ueta Y. Central effects of Ghrelin on appetite and fluid/water drinking behavior. Curr Protein Pept Sci. 2011;12(4):280-7. doi:10.2174/138920311795906727
51. van der Lely AJ, Tschöp M, Heiman ML, Ghigo E. Biological, physiological, pathophysiological, and pharmacological aspects of Ghrelin. Endocr Rev. 2004;25(3):426-57. doi:10.1210/er.2002-0029
52. Harvey RE, Howard VG, Lemus MB, Jois T, Andrews ZB, Sleeman MW. The ghrelin/GOAT system regulates obesity-induced inflammation in male mice. Endocrinology. 2017;158(7):2179-89. doi:10.1210/en.2016-1832
53. Mabudi H, Jamili S, Majd NE, Vosoughi G, Fatemi MR, Rashed S. The effects of Ghrelin on ovary histology in Barbus sharpeyi. J Anim Physiol Anim Nutr. 2011;95(5):599-602. doi:10.1111/j.1439-0396.2010.01089.x
54. Rajeswari JJ, Hatef A, Golshan M, Alavi SMH, Unniappan S. Metabolic stress leads to divergent changes in the ghrelinergic system in goldfish (Carassius auratus) gonads. Comp Biochem Physiol A Mol Integr Physiol. 2019;235:112-20. doi:10.1016/j.cbpa.2019.05.027
55. Sokołowska-Mikołajczyk M, Socha M, Szczerbik P, Epler P. The effects of Ghrelin on the in vitro spontaneous and sGnRH-A stimulated luteinizing hormone (LH) release from the pituitary cells of common carp (Cyprinus carpio L.). Comp Biochem Physiol A Mol Integr Physiol. 2009;153(4):386-90. doi:10.1016/j.cbpa.2009.03.012
56. Grey CL, Grayfer L, Belosevic M, Chang JP. Ghrelin stimulation of gonadotropin (LH) release from goldfish pituitary cells: presence of the growth hormone secretagogue receptor (GHS-R1a) and involvement of voltage-sensitive Ca2+ channels. Mol Cell Endocrinol. 2010;317(1):64-77. doi:10.1016/j.mce.2009.12.024
57. Navarro-Guillén C, Dias J, Rocha F, Castanheira MF, Martins CIM, Laizé V, et al. Does a ghrelin stimulus during zebrafish embryonic stage modulate its performance on the long-term? Comp Biochem Physiol A Mol Integr Physiol. 2019;228:1-8. doi:10.1016/j.cbpa.2018.10.019
58. Kang KS, Yahashi S, Matsuda K. The effects of Ghrelin on energy balance and psychomotor activity in a goldfish model: an overview. Int J Pept. 2011;2011:171034. doi:10.1155/2011/171034
59. Kang KS, Yahashi S, Matsuda K. Central and peripheral effects of Ghrelin on energy balance, food intake and lipid metabolism in teleost fish. Peptides. 2011;32(11):2242-7. doi:10.1016/j.peptides.2011.05.006
60. Miandare HK, Farahmand H, Ramezanpour S, Nematollahi MA, Rafiee G, Amiri BM. Effects of Ghrelin on some plasma hormonal changes in juvenile Persian sturgeon (Acipenser persicus). Comp Clin Pathol. 2012;21(5):1099-102. doi:10.1007/s00580-011-1239-y
61. Cruz SA, Tseng YC, Kaiya H, Hwang PP. Ghrelin affects carbohydrate-glycogen metabolism via insulin inhibition and glucagon stimulation in the zebrafish (Danio rerio) brain. Comp Biochem Physiol A Mol Integr Physiol. 2010;156(2):190-200. doi:10.1016/j.cbpa.2010.01.019
62. Blanco AM, Bertucci JI, Ramesh N, Delgado MJ, Valenciano AI, Unniappan S. Ghrelin facilitates GLUT2-, SGLT1- and SGLT2-mediated intestinal glucose transport in goldfish (Carassius auratus). Sci Rep. 2017;7:45024. doi:10.1038/srep45024
63. Velasco C, Librán-Pérez M, Otero-Rodiño C, López-Patiño MA, Míguez JM, Cerdá-Reverter JM, et al. Ghrelin modulates hypothalamic fatty acid-sensing and control of food intake in rainbow trout. J Endocrinol. 2016;228(1):25-37. doi:10.1530/JOE-15-0391
64. Salmerón C, Johansson M, Asaad M, Angotzi AR, Rønnestad I, Stefansson SO, et al. Roles of leptin and Ghrelin in adipogenesis and lipid metabolism of rainbow trout adipocytes in vitro. Comp Biochem Physiol A Mol Integr Physiol. 2015;188:40-8. doi:10.1016/j.cbpa.2015.06.017
65. Ma Y, Zhang H, Guo W, Yu L. Potential role of Ghrelin in the regulation of inflammation. FASEB J. 2022;36(9):e22508. doi:10.1096/fj.202200634R
66. Naseri F, Sirati-Sabet M, Sarlaki F, Keimasi M, Mokarram P, Siri M, et al. Ghrelin regulates crosstalk between apoptosis, necroptosis and autophagy programmed cell death pathways in the hippocampal neurons of amyloid-β 1–42-induced rat model of Alzheimer's disease. Res Square. 2022. doi:10.1007/s10989-022-10457-3
67. Shirshev SV, Nekrasova IV, Orlova EG, Gorbunova OL. Effects of leptin and Ghrelin on the expression of membrane molecules and cytokine production by NK cells from the peripheral blood. Biochem Mosc Suppl Ser Membr Cell Biol. 2017;11(1):54-61. doi:10.1134/S199074781604019X
68. Zhang X, Zeng Z, Liu Y, Liu D. Emerging relevance of Ghrelin in programmed cell death and its application in diseases. Int J Mol Sci. 2023;24(24):17254. doi:10.3390/ijms242417254
69. Smith RG, Jiang H, Sun Y. Developments in ghrelin biology and potential clinical relevance. Trends Endocrinol Metab. 2005;16(9):436-42. doi:10.1016/j.tem.2005.09.004
70. Esteban MÁ, Cuesta A, Chaves-Pozo E, Meseguer J. Phagocytosis in teleosts: implications of the new cells involved. Biology. 2015;4(4):907-22. doi:10.3390/biology4040907
71. Li B, Zeng M, Zheng H, Huang C, He W, Lu G, et al. Effects of Ghrelin on the apoptosis of human neutrophils in vitro. Int J Mol Med. 2016;38(3):794-802. doi:10.3892/ijmm.2016.2668
72. Tümer C, Bilgin HM, Obay BD, Diken H, Taşdemir E, Şermet A. Effect of ghrelin administration on phagocytic activity in acute cold-restraint stress exposed rats. Regul Pept. 2007;138(2):113-7. doi:10.1016/j.regpep.2006.08.010
73. Corrêa da Silva F, Aguiar C, Pereira JAS, de Brito Monteiro L, Davanzo GG, Codo AC, et al. Ghrelin effects on mitochondrial fitness modulates macrophage function. Free Radic Biol Med. 2019;145:61-6. doi:10.1016/j.freeradbiomed.2019.09.012
74. Peterson BC, Small BC, Bilodeau L. Effects of GH on immune and endocrine responses of channel catfish challenged with Edwardsiella ictaluri. Comp Biochem Physiol A Mol Integr Physiol. 2007;146(1):47-53. doi:10.1016/j.cbpa.2006.08.042
75. Sakai M, Kobayashi M, Kawauchi H. In vitro activation of fish phagocytic cells by GH, prolactin and somatolactin. J Endocrinol. 1996;151(1):113-8. doi:10.1677/joe.0.1510113
76. Yada T, Kaiya H, Mutoh K, Azuma T, Hyodo S, Kangawa K. Ghrelin stimulates phagocytosis and superoxide production in fish leukocytes. J Endocrinol. 2006;189(1):57-65. doi:10.1677/joe.1.06187
77. Liu C, Chu D, Kalantar-Zadeh K, George J, Young HA, Liu G. Cytokines: from clinical significance to quantification. Adv Sci. 2021;8(15):2004433. doi:10.1002/advs.202004433
78. Chang RJ, Wang HL, Qin MB, Liang ZH, He JP, Wei YL, et al. Ghrelin inhibits IKKβ/NF-κB activation and reduces proinflammatory cytokine production in pancreatic acinar AR42J cells treated with cerulein. Hepatobiliary Pancreat Dis Int. 2021;20(4):366-75. doi:10.1016/j.hbpd.2020.05.004
79. Beynon AL, Brown MR, Wright R, Rees MI, Sheldon IM, Davies JS. Ghrelin inhibits LPS-induced release of IL-6 from mouse dopaminergic neurones. J Neuroinflammation. 2013;10:40. doi:10.1186/1742-2094-10-40
80. Jafari A, Sadeghpour S, Ghasemnejad-Berenji H, Pashapour S, Ghasemnejad-Berenji M. Potential antioxidative, anti-inflammatory and immunomodulatory effects of Ghrelin, an endogenous peptide from the stomach in SARS-CoV2 infection. Int J Pept Res Ther. 2021;27(3):1875-83. doi:10.1007/s10989-021-10217-9
81. Xia Q, Pang W, Pan H, Zheng Y, Kang JS, Zhu SG. Effects of Ghrelin on the proliferation and secretion of splenic T lymphocytes in mice. Regul Pept. 2004;122(3):173-8. doi:10.1016/j.regpep.2004.06.016
82. Sakai M, Hikima J, Kono T. Fish cytokines: current research and applications. Fish Sci. 2021;87(1):1-9. doi:10.1007/s12562-020-01476-4
83. Shved N, Berishvili G, Mazel P, Baroiller JF, Eppler E. Growth hormone (GH) treatment acts on the endocrine and autocrine/paracrine GH/IGF-axis and on TNF-α expression in bony fish pituitary and immune organs. Fish Shellfish Immunol. 2011;31(6):944-52. doi:10.1016/j.fsi.2011.08.012
84. Kono T, Kitao Y, Sonoda K, Nomoto R, Mekata T, Sakai M. Identification and expression analysis of ghrelin gene in common carp Cyprinus carpio. Fish Sci. 2008;74(3):603-12. doi:10.1111/j.1444-2906.2008.01564.x
85. Dixit VD, Schaffer EM, Pyle RS, Collins GD, Sakthivel SK, Palaniappan R, et al. Ghrelin inhibits leptin- and activation-induced proinflammatory cytokine expression by human monocytes and T cells. J Clin Invest. 2004;114(1):57-66. doi:10.1172/JCI21134
86. Koo GC, Huang C, Camacho R, Trainor C, Blake JT, Sirotina-Meisher A, et al. Immune enhancing effect of a growth hormone secretagogue. J Immunol. 2001;166(6):4195-201. doi:10.4049/jimmunol.166.6.4195
87. Zhou M, Aziz M, Ochani M, Yang WL, Sharma A, Wang P. The protective role of human Ghrelin in sepsis: restoration of CD4 T cell proliferation. PLoS One. 2018;13(7):e0201139. doi:10.1371/journal.pone.0201139
88. Di Giovangiulio M, Stakenborg N, Bosmans G, Meroni E, Farro G, Gomez-Pinilla PJ, et al. Ghrelin receptor modulates T helper cells during intestinal inflammation. Neurogastroenterol Motil. 2015;27(11):1542-52. doi:10.1111/nmo.12640
89. Batista CR, Figueiredo MA, Almeida DV, Romano LA, Marins LF. Effects of somatotrophic axis (GH/GHR) double transgenesis on structural and molecular aspects of the zebrafish immune system. Fish Shellfish Immunol. 2015;45(2):725-32. doi:10.1016/j.fsi.2015.05.034
90. Chorny A, Anderson P, Gonzalez-Rey E, Delgado M. Ghrelin protects against experimental sepsis by inhibiting high-mobility group box 1 release and by killing bacteria. J Immunol. 2008;180(12):8369-77. doi:10.4049/jimmunol.180.12.8369
91. Xiao Y, Jin L, Zhang C. From a hunger-regulating hormone to an antimicrobial peptide: gastrointestinal derived circulating endocrine hormone-peptide YY exerts exocrine antimicrobial effects against selective gut microbiota. Gut Microbes. 2024;16(1):2316927. doi:10.1080/19490976.2024.2316927
92. Min C, Ohta K, Kajiya M, Zhu T, Sharma K, Shin J, et al. The antimicrobial activity of the appetite peptide hormone ghrelin. Peptides. 2012;36(2):151-6. doi:10.1016/j.peptides.2012.05.006
93. van Muilekom DR, Collet B, Rebl H, Zlatina K, Sarais F, Goldammer T, Rebl A. Lost and found: the family of NF-κB inhibitors is larger than assumed in salmonid fish. Int J Mol Sci. 2023;24(12):10229. doi:10.3390/ijms241210229
94. Zhu J, Zhou T, Menggen M, Aimulajiang K, Wen H. Ghrelin regulating liver activity and its potential effects on liver fibrosis and Echinococcosis. Front Cell Infect Microbiol. 2024;13:1324134. doi:10.3389/fcimb.2023.1324134
95. Gao F, Zhao Y, Shi X, Qiao D, Pei C, Kong X. Signalling regulation of reactive oxygen species in fish inflammation. Rev Aquac. 2024;16(3):1266-85. doi:10.1111/raq.12895
96. Bian DD, Zhang X, Zhu XR, Tang WH, Peng Q, Chen YH, et al. The Nrf2-Keap1/ARE signaling pathway in aquatic animals. Int J Biol Macromol. 2025;254:142595. doi:10.1016/j.ijbiomac.2025.142595
97. Olavarría VH, Sepulcre MP, Figueroa JE, Mulero V. Prolactin-induced production of reactive oxygen species and IL-1β in leukocytes from the bony fish gilthead seabream involves Jak/Stat and NF-κB signaling pathways. J Immunol. 2010;185(7):3873-83. doi:10.4049/jimmunol.0902306
98. Philip AM, Vijayan MM. Stress-immune-growth interactions: cortisol modulates suppressors of cytokine signaling and JAK/STAT pathway in rainbow trout liver. PLoS One. 2015;10(6):e0129299. doi:10.1371/journal.pone.0129299
99. Liongue C, O'Sullivan LA, Trengove MC, Ward AC. Evolution of JAK-STAT pathway components: mechanisms and role in immune system development. PLoS One. 2012;7(3):e32777. doi:10.1371/journal.pone.0032777
100. Ishida J, Saitoh M, Ebner N, Springer J, Anker SD, von Haehling S. Growth hormone secretagogues: history, mechanism of action, and clinical development. JCSM Rapid Commun. 2020;3(1):25-37. doi:10.1002/rco2.9
101. Bowers CY, Momany FA, Reynolds GA, Hong A. On the in vitro and in vivo activity of a new synthetic hexapeptide that acts on the pituitary to specifically release growth hormone. Endocrinology. 1984;114(5):1537-45. doi:10.1210/endo-114-5-1537
102. Cibrián D, Ajamieh H, Berlanga J, León OS, Alba JS, Kim MJT, et al. Use of growth-hormone-releasing peptide-6 (GHRP-6) for the prevention of multiple organ failure. Clin Sci. 2006;110(5):563-73. doi:10.1042/CS20050374
103. Berlanga-Acosta J, Cibrian D, Valiente-Mustelier J, Suárez-Alba J, García-Ojalvo A, Falcón-Cama V, et al. Growth hormone releasing peptide-6 (GHRP-6) prevents doxorubicin-induced myocardial and extra-myocardial damages by activating prosurvival mechanisms. Front Pharmacol. 2024;15:1402138. doi:10.3389/fphar.2024.1402138
104. Martínez R, Carpio Y, Arenal A, Lugo JM, Morales R, Martín L, et al. Significant improvement of shrimp growth performance by growth hormone-releasing peptide-6 immersion treatments. Aquac Res. 2017;48(9):4632-45. doi:10.1111/are.13286
105. Berlanga-Acosta J, Abreu-Cruz A, Barco Herrera DG, Mendoza-Marí Y, Rodríguez-Ulloa A, García-Ojalvo A, et al. Synthetic growth hormone-releasing peptides (GHRPs): a historical appraisal of the evidences supporting their cytoprotective effects. Clin Med Insights Cardiol. 2017;11:1179546817694558. doi:10.1177/1179546817694558
106. Martinez R, Carpio Y, Morales A, Lugo JM, Herrera F, Zaldívar C, et al. Oral administration of the growth hormone secretagogue-6 (GHRP-6) enhances growth and non-specific immune responses in tilapia (Oreochromis sp.). Aquaculture. 2016;452:304-10. doi:10.1016/j.aquaculture.2015.11.014
107. Hernández L, Camacho H, Nuñez-Robainas A, Palenzuela DO, Morales A, Basabe L, et al. Growth hormone secretagogue peptide-6 enhances oreochromicins transcription and antimicrobial activity in tilapia (Oreochromis sp.). Fish Shellfish Immunol. 2021;119:508-15. doi:10.1016/j.fsi.2021.08.011
108. Rodríguez-Viera L, Martí I, Martínez R, Perera E, Estrada MP, Mancera JM, Martos-Sitcha JA. Feed supplementation with the GHRP-6 peptide, a ghrelin analog, improves feed intake, growth performance and aerobic metabolism in the gilthead sea bream Sparus aurata. Fishes. 2022;7(1):31. doi:10.3390/fishes7010031
109. Martinez R, Gonzalez K, Gonzalez A, Ubieta K, Herrera F, Reyes O, et al. Biological activity of a new growth hormone secretagogue: study in fish and murine cell line. J Biotechnol Biomater. 2016;1(3):5. doi:10.4172/2155-952X.1000e113
110. Martinez R, Ubieta K, Herrera F, Forellat A, Morales R, de la Nuez A, et al. A novel GH secretagogue, A233, exhibits enhanced growth activity and innate immune system stimulation in teleosts fish. J Endocrinol. 2012;214(3):409-19. doi:10.1530/JOE-11-0373
111. Martinez R, Fernández-Trujillo MA, Hernández L, Page A, Béjar J, Estrada MP. Growth hormone secretagogue peptide A233 upregulates Mx expression in teleost fish in vitro and in vivo. Arch Virol. 2022;167(10):2041-7. doi:10.1007/s00705-022-05504-9
112. Mishra SS, Das R, Choudhary P, Debbarma J, Sahoo SN. Present status of fisheries and impact of emerging diseases of fish and shellfish in Indian aquaculture. J Aquat Res Mar Sci. 2017;5:5-26. doi:10.29199/ARMS.2017.03
113. Vignesh R, Karthikeyan BS, Periyasamy N, Devanathan K. Antibiotics in aquaculture: an overview. South Asian J Exp Biol. 2011;1(3):114-20.
Received: February 14, 2024 / Accepted: May 4, 2025 / Published: June 15, 2025
Citation: Reyes D, Estrada MP, Martínez R. Ghrelin as a promising immunostimulant in aquaculture: mechanisms and therapeutic potential. Bionatura. 2025;2(2):6. doi:10.70099/BJ/2025.02.02.6.
Additional information Correspondence should be addressed to olinda.nunez@unah.edu.hn
Peer review information. Bionatura thanks anonymous reviewer(s) for their contribution to the peer review of this work using https://reviewerlocator.webofscience.com/
ISSN.3020-7886
All articles published by Bionatura Journal are made freely and permanently accessible online immediately upon publication, without subscription charges or registration barriers.
Publisher's Note: Bionatura Journal stays neutral concerning jurisdictional claims in published maps and institutional affiliations.
Copyright: © 2025 by the authors. They were submitted for possible open-access publication under the terms and conditions of the Creative Commons Attribution (CC BY) license (https://creativecommons.org/licenses/by/4.0/).